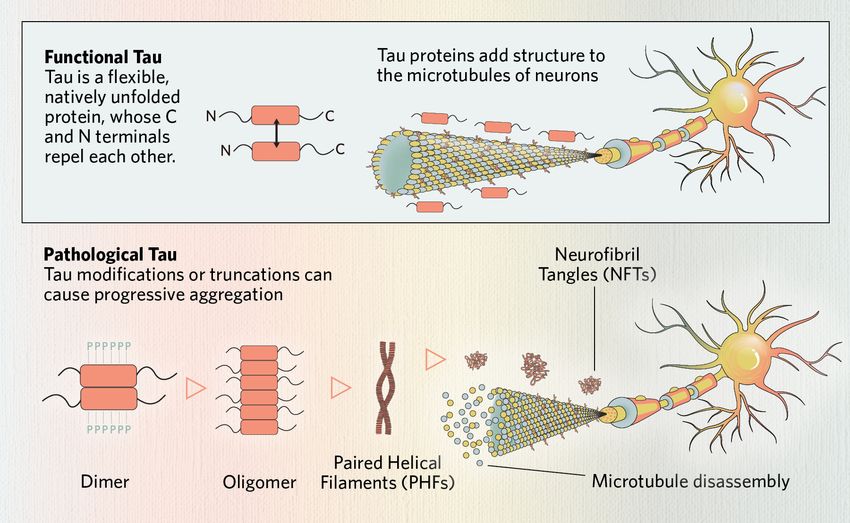
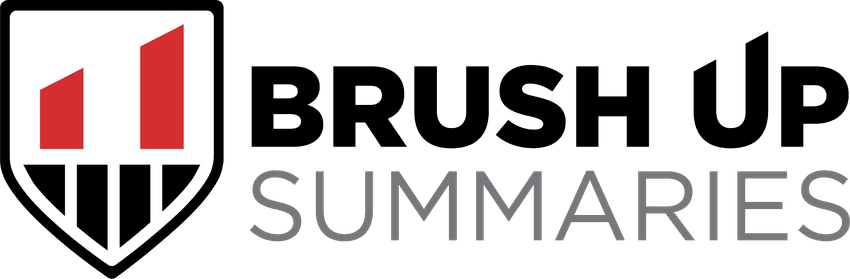
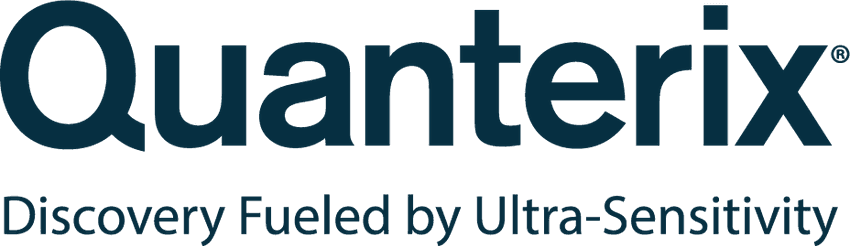
This Brush Up is sponsored by Quanterix. Learn more about tau biomarkers and clinical applications.
Tau is a flexible protein that stabilizes the internal structure of neurons and supports intracellular transport.1 When dysregulated, tau becomes prone to misfolding and aggregation, driving a class of neurodegenerative diseases called tauopathies. This article explores tau’s physiological roles, structural complexity, and its transformation into a pathological state, which has relevance to disease research and biomarker breakthroughs.
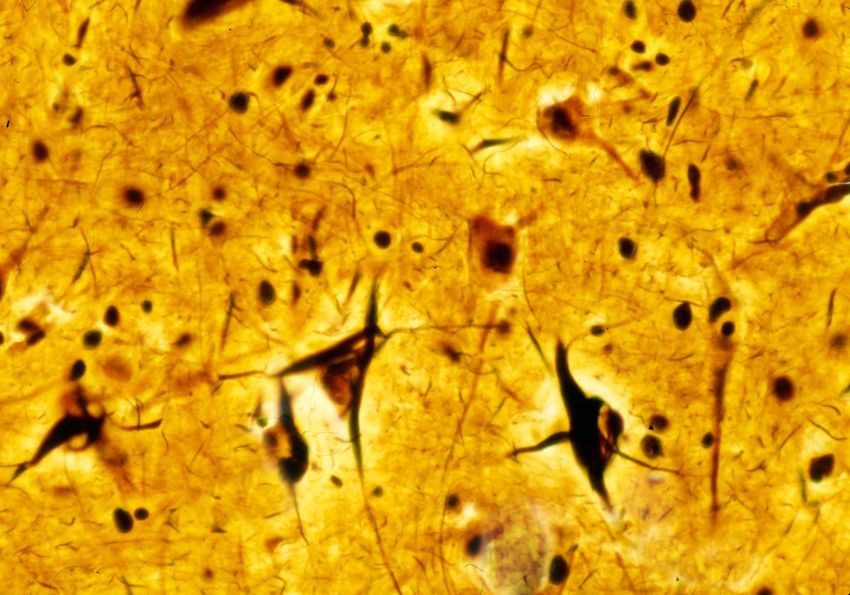
Researchers study tau protein to understand its role in maintaining neuronal stability and its pathological transformation into neurofibrillary tangles. These tangles, visible in the cortex of Alzheimer’s disease brains, are a defining tauopathy feature and correlate with cognitive decline.
iStock, JOSE LUIS CALVO MARTIN & JOSE ENRIQUE GARCIA-MAURIÑO MUZQUIZ
What Is Tau Protein?
Tau protein is a microtubule-associated protein (MAP) encoded by the MAPT gene.1 Tau is primarily localized in axons, where it binds, compresses, and stabilizes polymerized microtubules.1-3 Microtubule structures are critical for organelle and synaptic component axonal transport within neurons.1 Tau regulates this axonal transport through multiple mechanisms via the motor proteins dynein and kinesin.1
Tau protein also contributes to synaptic plasticity and modulates intracellular signaling pathways, particularly through its dynamic association with tubulin and plasma membranes.1 In addition to its cytoplasmic roles, tau localizes to the nucleus, where it protects DNA and RNA from oxidative stress and helps maintain chromatin stability.4 Tau’s localization and function are regulated by specific modifications and conformational flexibility, which also render it susceptible to misfolding under pathological conditions.1
Tau Structure, Isoforms, and Localization
Tau’s structural diversity arises from alternative splicing of the MAPT gene, which produces six isoforms that differ in the number of amino (N)-terminal inserts and microtubule-binding repeat domains—either three (3R) or four (4R) repeats.1,5 The balance between 3R and 4R isoforms is tightly regulated in the adult human brain, and deviations from this ratio are associated with distinct tauopathies.6 MAPT mutations and transcript mis-splicing can cause tau dysfunction.1
While tau is enriched in axons under physiological conditions, scientists characterize disease states by the protein’s redistribution to dendrites, the soma, and synaptic terminals. Tau’s dissociation from microtubules leads to mislocalization that impairs intracellular and nuclear pore transport, synaptic function, and cytoskeletal organization.1,7,8
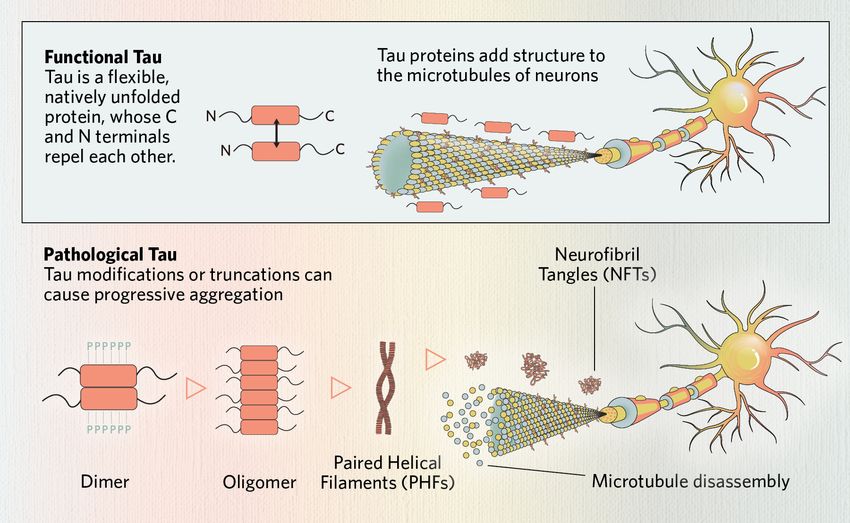
Tau protein is an essential component to neuronal function. Tau dysfunction often begins with disrupted microtubule-binding, which destabilizes microtubules, impairing neuronal structure and transport. Modified or truncated tau monomers can form dimers, which assemble into soluble oligomers. These oligomers further align into paired helical filaments (PHFs), the structural core of neurofibrillary tangles (NFTs)—a defining pathological feature of tauopathies.
Modified from ©istock.com, ttsz; Designed by Alisha Vroom
Tau Post-translational Modifications and Dysregulation
Post-translational modifications (PTMs) regulate tau’s interactions with tubulin, subcellular localization, and degradation, shaping both its physiological function and its potential to undergo pathological transformation.1,9,10
- Phosphorylation is the most studied PTM and involves adding negatively charged phosphate groups to the protein, primarily to tau’s carboxyl (C)-terminal domain.1 In disease, tau hyperphosphorylation reduces its microtubule binding affinity, degradation, and ability to protect DNA.1,11
- Truncation, observed in Alzheimer’s disease (AD), Pick’s disease (PiD), Parkinson’s disease (PD), and vascular disease, by proteolytic cleavage generates aggregation-prone fragments that misfold more readily than full-length tau protein.1,5,10,11
- Ubiquitination normally targets tau aggregates for removal, but the ubiquitin-proteasome system (UPS) is impaired in tauopathies, contributing to toxic protein buildup.5
- Acetylation blocks tau’s lysine residues that are typically ubiquitination sites required for proteasomal degradation, resulting in intracellular tau accumulation, notably in AD, PiD, and frontotemporal lobar degeneration with tau inclusions (FTLD-tau).12
Together, these and other PTMs alter tau’s microtubule-binding capacity, facilitating its conversion into aggregation-prone, neurotoxic forms. The PTM patterns seen within tauopathies such as AD are heterogenous, suggesting that there is no specific PTM combination responsible for inducing a disease state.13
Tau Pathologies and Disease Progression
Tau aggregation in the brain is a central feature of neurodegenerative tauopathies that correlates with neuronal loss, synaptic dysfunction, and cognitive decline.8
In pathological states, tau undergoes conformational changes, forming dimers and oligomers, which further assemble into paired helical filaments (PHFs) and neurofibrillary tangles (NFTs).5 Tau aggregates resist degradation by proteolysis, and their structural properties can vary depending on MAPT mutations, isoform composition, and PTMs.1,6,14 Emerging research indicates that distinct tauopathies may be associated with specific conformational variants—or “strains“—of aggregated tau.14
Tauopathies can be categorized based on whether tau aggregation is a primary driver or a secondary consequence of neurodegeneration.
While NFTs are a pathological hallmark, accumulating evidence suggests that highly phosphorylated soluble oligomers are more closely linked to neurotoxicity and symptom onset.9,16 Prion-like tau oligomer propagation or seeding along synaptically connected networks may underlie the stereotypical pathological progression observed in tauopathies, although the precise mechanisms remain unknown.1,14,16 Scientists have also linked dysregulated tau expression to disease outside the brain, including cancer, cardiovascular disorders, and retinal degeneration, broadening its clinical relevance.11
Future Directions and Research Gaps
Targeting tau
Ongoing efforts to develop tau-based therapeutics focus on preventing, reversing, or modulating the protein’s pathological forms and behavior. Researchers are currently investigating several molecular mechanisms.
- PTM regulation: Targeting enzymes that mediate tau phosphorylation, acetylation, and truncation is a priority, though causal relationships between these modifications and disease remain unexplained.5,13,17
- Tau aggregation inhibitors: Compounds that block oligomer and fibril formation are advancing through preclinical pipelines but have yet to demonstrate clinical efficacy.17,18
- Immunotherapy: Antibody-based therapies are being developed in the hopes of neutralizing oligomeric tau and its propagation between neurons.5,15,17
- Propagation and uptake: Strategies that disrupt intercellular transfer, cellular internalization, and tau’s prion-like spread are emerging research areas.16,19
- Microtubule stabilization: Stabilizing agents aim to compensate for tau dysfunction in maintaining axonal integrity.15,17
- Tau clearance: Scientists are researching how to enhance degradation through the UPS and autophagy-lysosome system.17
While primary causes of tau dysfunction are not yet known, some evidence suggests that cytokines and neuroinflammation promote tau pathology.20,21 Future research might target inflammatory pathways or microglia to prevent and clear misfolded tau.20,22
Tau biomarkers
Improving diagnostic biomarkers remains a critical focus. In individuals with AD, exosomes containing tau oligomers can travel beyond the originating neuron, into the extracellular space, cerebrospinal fluid (CSF), and blood serum.16 Clinicians have traditionally measured tau levels in CSF during clinical trials to monitor disease state and therapeutic response, but scientists are developing more precise tools for detecting and tracking pathology.11,15
Advances in tau biomarker detection have introduced several technological platforms. Tau positron emission tomography (Tau-PET) imaging remains the gold standard for confirming neurofibrillary tangle pathology in vivo, but its high cost and limited accessibility constrain its use for routine screening or longitudinal monitoring. Mass spectrometry-based approaches, such as immunoprecipitation mass spectrometry (IP-MS), provide high sensitivity and specificity for plasma tau detection but are limited by complexity and cost. In contrast, immunoassay-based methods offer a more scalable solution. Ultra-sensitive platforms such as the Simoa® digital immunoassay technology have reshaped tau biomarker research by enabling precise measurement of low-abundance tau species in blood, serum, and plasma.
Researchers have identified promising plasma-based biomarkers for early detection and disease staging, including phosphorylated tau at threonine 217 (p-Tau217), microtubule-binding region tau 243 (MTBR-Tau243), and brain-derived tau (BD-Tau). These new biomarkers, combined with advanced detection technologies that capture biomarkers at concentrations well below conventional detection limits, are accelerating both research and clinical applications.
- Guo T, et al. Roles of tau protein in health and disease. Acta Neuropathol. 2017;133(5):665-704.
- Dehmelt L, Halpain S. The MAP2/Tau family of microtubule-associated proteins. Genome Biol. 2005;6(1):204.
- Siahaan V, et al. Microtubule lattic3e spacing governs cohesive envelope formation of tau family proteins. Nat Chem Biol. 2022;18(11):1224-1235.
- Violet M, et al. A major role for Tau in neuronal DNA and RNA protection in vivo under physiological and hyperthermic conditions. Front Cell Neurosci. 2014;8:84.
- Chong FP, et al. Tau proteins and tauopathies in Alzheimer’s disease. Cell Mole Neurobiol. 2018;38(5):965-980.
- de Silva R, et al. An immunohistochemical study of cases of sporadic and inherited frontotemporal lobar degeneration using 3R- and 4R-specific tau monoclonal antibodies. Acta Neuropathol. 2006;111(4):329-340.
- Eftekharzadeh B, et al. Tau protein disrupts nucleocytoplasmic transport in Alzheimer’s disease.Neuron. 2018;99(5):925-940.e927.
- Hoover BR, et al. Tau mislocalization to dendritic spines mediates synaptic dysfunction independently of neurodegeneration. Neuron. 2010;68(6):1067-1081.
- Cowan CM, Mudher A. Are tau aggregates toxic or protective in tauopathies?Front Neurol. 2013;4.
- Kolarova M, et al. Structure and pathology of tau protein in Alzheimer disease. Int J Alzheimers Dis. 2012;2012:731526.
- Abyadeh M, et al. Amyloid-beta and tau protein beyond Alzheimer’s disease. Neural Regen Res. 2024;19(6):1262-1276.
- Min S-W, et al. Acetylation of tau inhibits its degradation and contributes to tauopathy. Neuron. 2010;67(6):953-966.
- Wegmann S, et al. A current view on tau protein phosphorylation in Alzheimer’s disease. Curr Opin Neurobiol. 2021;69:131-138.
- Sanders David W, et al. Distinct tau prion strains propagate in cells and mice and define different tauopathies. Neuron. 2014;82(6):1271-1288.
- Masnata M, et al. Targeting tau to treat clinical features of Huntington’s disease. Front Neurol. 2020;11:580732.
- Jackson NA, et al. The prion-like transmission of tau oligomers via exosomes. Front Aging Neurosci. 2022;14:974414.
- Muralidar S, et al. Role of tau protein in Alzheimer’s disease: The prime pathological player. Int J Biol Macromol. 2020;163:1599-1617.
- Imbimbo BP, et al. A critical appraisal of tau-targeting therapies for primary and secondary tauopathies. Alzheimers Dement. 2022;18(5):1008-1037.
- Holmes BB, Diamond MI. Prion-like properties of tau protein: The importance of extracellular tau as a therapeutic target. J Biol Chem. 2014;289(29):19855-19861.
- Zilka N, et al. Who fans the flames of Alzheimer’s disease brains? Misfolded tau on the crossroad of neurodegenerative and inflammatory pathways. J Neuroinflammation. 2012;9(1):47.
- Kovac A, et al. Misfolded truncated protein Ï„ induces innate immune response via MAPK pathway. J Immun. 2011;187(5):2732-2739.
- Gao C, et al. Microglia in neurodegenerative diseases: Mechanism and potential therapeutic targets. Signal Transduct Target Ther. 2023;8(1):359.
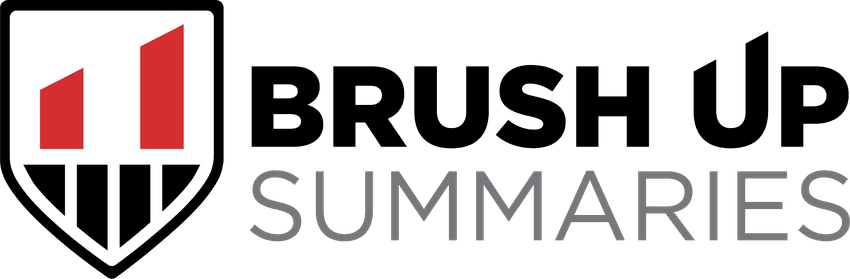
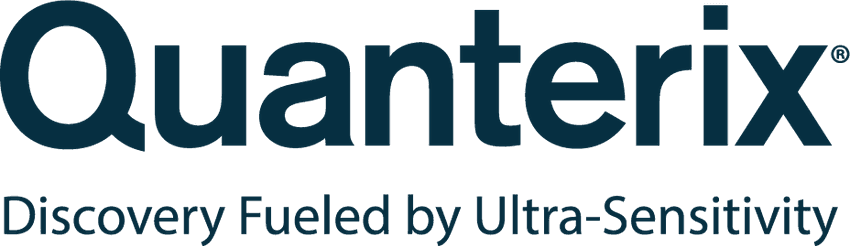
Source link